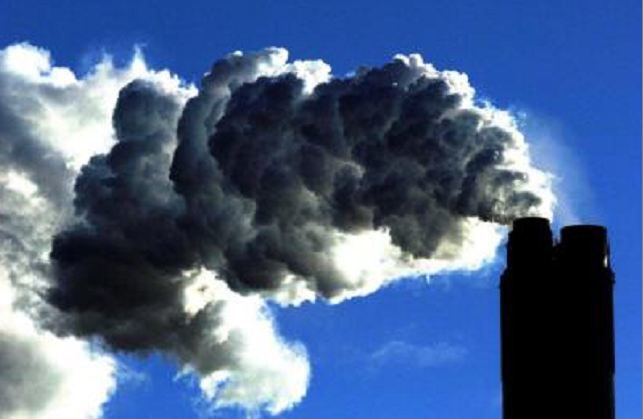
It has been encouraging to witness many oil and gas majors sign up to the energy transition and net zero. Repsol, BP, Total, Shell and Equinor have all outlined their strategies.
Common themes amongst the majors are:
- Operational energy efficiency
- Shift from oil to gas
- Hydrogen
- Renewables
- Bio fuels
- CO2 sinks (land use)
- CCS
I have always considered Norway to be best in class when it comes to environmental management. It is therefore not surprising that Equinor claim to have the best metric for operational energy efficiency as reflected in its CO2 intensity metric. Equinor’s Climate Road Map includes the following chart.
In their climate roadmap, Equinor also state “Equinor already has one of the industry’s lowest upstream carbon intensities and aims to reduce this further. We are moving forward the 2030 ambition of <8 kg CO₂ per barrel of oil equivalent (boe) to 2025. The current global industry average is 18 kg CO₂ per boe (IOGP).” Note that gas production can be measured in boe; it is the volume of gas that has the same thermal energy as a barrel of oil.
Energy intensity is a measure of the amount of CO2 embedded in the energy required to produce a barrel of oil or its equivalent. In upstream operations energy is required for pumping, gas compression, gas treatment, cooling systems, heating systems, supporting the accommodation block, drilling, lighting etc. Providing that energy produces CO2. The energy intensity also includes the CO2 produced from gas flaring.
The required energy associated with upstream installations is commonly supplied by burning gas in a turbine. The rotational energy of the turbine is used to generate electricity via an alternator. A small version of an onshore gas fired power station.
The CO₂ produced from burning gas for energy and from flaring can be calculated for any given installation. Dividing the CO2 produced by the boe production rate yields the kg CO₂ per boe metric.
It is though important that this kg CO₂ per boe metric is put in context.
Firstly, if a barrel of oil is used as fuel, the associated emissions will be around 400 kg CO₂. Hence, using the IOGP upstream average of 18 kg CO₂ per boe, and including the fate of the oil (combustion), the overall metric for oil production and use would be 418 kg CO₂ per boe. Reducing the average upstream intensity by 55% to 8 kg CO₂ per boe could be delivered if oil consumption is reduced by 2.5%. It is therefore clear that reducing the amount of oil used as fuel will have a much larger impact towards achieving net zero than improvements in operational energy efficiency.
Secondly, the average global industry metric of 18 kg CO₂ per boe makes no recognition of the type of hydrocarbon field and the field timeline. This is particularly the case for oil fields where it is common that dry oil is produced in the first few years followed by oil production being displaced by an increasing quantity of water. Towards the end of on oilfield’s operational life many production wells will be flowing with 5% oil and 95% water. Moving water in upstream operations is highly energy intensive.
As will be demonstrated using average kg CO₂ per boe can be misleading when quantifying the energy efficiency of an upstream asset.
Consider a typical oil field conditioning oil and gas for onward transportation. To meet transportation specifications, the gas is compressed to high pressures to allow for transfer to shore. Gas compression is the largest energy consumer within upstream operations.
Similarly oil is typically pumped to an onshore terminal. Distances are often hundreds of kilometres hence pumping energy requirement can be very significant.
To maximise hydrocarbon recovery oil, it is common practice to utilise waterflood. Water is injected into the rock structure to push and sweep oil to the production wells. Large volumes of water are required at high pressures thus water injection is also an energy intensive process. As an example, the Chirag Field in Azerbaijan has four 27 MW pumps capable of injecting 1 million barrels water/day at 480 bar. The power required for each pump equates to the electricity use of around 30,000 UK households.
Furthermore, as water breaks into the production wells, restraining forces due to the heavier water are increased thus constraining oil production. To minimise this restraining effect, it is common oilfield practice to inject gas into the production well to lower the weight of the fluid. This is known as gas lift. Gas lift rates increase with increasing water production and compressing the required lift gas is energy intensive.
Let’s test the metric out on two identical oilfields designed for 100,000 barrels of oil per day (bopd) at differing operational time lines.
Field A at year 2, where oil is at oil design rates and water is not being produced with the oil. Gas production is 9600 boed. Water injection rates are 120,000 bpd
Field B at year 20, where oil production rates have dropped to 10,000 bopd with produced water rates at 130,000 bpd. Gas production is 960 boed. Water injection rates have increased to 150,000 bpd.
Both fields use produced gas in gas turbines to generate electricity for the energy users.
Field A
The total electrical energy requirement is 25.9 MW, this is supplied by an open cycle gas turbine that is 35% efficient – 35% of the energy in the fuel gas is converted to electricity.
For the given energy requirement of 25.9MW, the energy intensity metric for the power generation plant is calculated as 3.2 kg CO₂ per boe.
Field A has an average flare rate that corresponds to 0.7 kg CO₂ per boe.
Hence the combined intensity metric for Field A is 3.9 kg CO₂/boe. On the face of it a very good metric compared to the industry average of 18 kg CO₂/boe. Note that oil fields where no water is being produced generally exhibit very good metrics.
Field B
Total electrical energy requirement is 22.7 MW, in this instance supplied by a combined cycle gas turbine that is 50% efficient – 50% of the energy in the fuel gas is converted to electricity. Substantially more energy efficient than Field A. Field B also uses high efficiency pumps and compressors and, unlike Field A, deploys a flare gas recovery system.
For the given energy requirement of 22.7 MW the energy intensity metric for the power generation plant is calculated as 19.4 kg CO₂ per boe.
Despite deploying a flare gas recovery system and flaring less gas, the intensity metric for Field B flare is 3.5 kg CO₂ per boe. This is much higher than Field A as a consequence of the low oil production rate.
Hence the overall emissions metric for Field B is 22.9 kg CO₂ per boe. Poorer than the IOGP average despite deploying high energy efficient equipment.
Comparing the intensity metric for Field A and B it is clear that Field A has a substantially better kg CO₂ per boe metric. As stated above, Field B has deployed superior energy efficiency plant when compared to Field A – combined cycle generation, more efficient pumps and compressors and flare gas recovery. Field B metrics poorly since much of the energy required is used to move water – this is not uncommon for ageing oil fields.
It is therefore clear that the kg CO2 per boe overall field metric is not accurately representing the energy status of Field B. The metric is very misleading with respect to oilfield energy efficiency performance particularly for mature fields co-producing large quantities of water with the oil. The UK is a mature oilfield province hence many operating fields are producing oil together with high volumes of produced water. Whilst UK fields may be operating efficiently from an energy standpoint many will not score well utilizing the overall energy metric of kg CO2 per boe.
To compare operational oilfield facilities on a like for like basis requires examination of the level below the overall intensity metric. This would involve comparing the energy performance of the main systems – gas compression, pumping and power generation. A systems approach would be a far truer reflection of operational energy intensity than IOGP’s overall intensity metric.
There is much interest in LNG as an enabler to net zero. The CO2 intensity metric for LNG production is around 40 – 45 kg CO2 per boe. This is double that of the average upstream metric produced by IOGP and appears to cast a poor light on LNG. The overall benefit of LNG is seen when it is used as fuel to produce electricity or used in domestic or industrial heating. Using LNG as fuel has a much lower kg CO2 per boe when compared to burning any other fossil fuel. Hence the combined metric of LNG production with power and heat generation would be superior to producing and burning oil. It is another example of where using a metric and not considering the full context can be misleading.
As a footnote, the most energy efficient action that can be taken for upstream operations is to deploy combined cycle power generation. Hitherto, combined cycle offshore plant has been avoided due to cost and complexity. Recognising the oil major’s net zero plans it will be interesting to see how many new offshore oilfields will incorporate combined cycle power generation.
Tom Baxter is visiting professor of chemical engineering at Strathclyde University and a retired technical director at Genesis Oil and Gas Consultants
Recommended for you
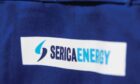